READ BY CHAPTER
Being the Change: Live Well and Spark a Climate Revolution
4. Global Warming: The Outlook
There’s already enough science to know what we need to do, and we’re not doing it.
—Ken Caldeira, climate scientist
In Chapter 3, I described how we know that human-emitted CO2 is the primary driver for the observed global warming trend. In this chapter, I’ll briefly describe some of the ramifications of this warming for humans and nonhumans. As before, I won’t attempt to be complete.1 My goals in doing so are to further describe what we know and what we don’t know, and to further clarify the likely duration of global warming.
Impacts
Global warming is bringing some benefits. Farmers and gardeners in cold regions have longer growing seasons and warmer soils; corporations will soon be able ship their products across an ice-free Arctic Ocean; death rates due to cold weather will likely decline.
In my opinion, though, the harmful impacts of global warming outweigh these benefits, and they will be permanent for all human purposes (as we’ll discuss below). If we decide we don’t like a warmer world, there will be no going back. The precautionary principle applies here, in spades.
Most of the impacts aren’t all-or-nothing. For most, costs will mount with temperature. Every degree of global warming will make human life on Earth more difficult.
Heat waves
The number of hot days and nights in a year have increased, and heat waves are more frequent and last longer.2 Europe’s 2003 heat wave, which killed 70,000 people,3 was the first individual event statistically attributed to global warming.4 In 2014, heat waves were already ten times as likely as they were just ten years earlier, and this frequency is increasing.5
Sixth mass extinction
Global warming, along with the sheer space taken up by so many humans, is driving an ongoing mass extinction event. Plants and animals are migrating to cooler habitats; those that can’t move, or move quickly enough, are vanishing. There are numerous scientific studies detailing the rapid migration of animals and plants pole- ward at an average rate of over a mile per year, and where possible to higher elevations.6 Marine species are also moving poleward, and doing so even more rapidly than land species.7
It has been estimated that, by 2050, global warming alone, depending on how aggressively we try to stop it, will commit 15% to 37% of all species to extinction.8 Interestingly, though, the fossil record from the time of the Paleocene-Eocene Thermal Maximum (PETM) global warming event does not show a corresponding mass extinction.9 This might be because warming happened 100 times more slowly in the PETM, giving species time to adapt.
In his book Half-Earth, biologist E. O. Wilson wrote, “Bio- diversity as a whole forms a shield protecting each of the species that together compose it, ourselves included…. As more and more species vanish or drop to near extinction, the rate of extinction of the survivors accelerates.”10
Today’s extinction rate is already about 1,000 times the background rate, and the extinction pressure from global warming is accelerating.11
Ocean acidification
Because a quarter of our CO2 emissions dissolve into the ocean and form carbonic acid, the ocean is becoming more acidic. Indeed, global mean ocean pH departed from its historical bounds in 2008; ocean dwellers are already living in a new world.12
Acidification and rising water temperatures have devastated the world’s coral reefs, a blow to global biodiversity. Other ocean ecosystems are also changing.
Destructive weather
Global warming is changing the hydrologic cycle. A warmer atmosphere holds more water, and the amount of precipitation over northern hemisphere land masses has increased.13 In some regions, including across the US, this increased precipitation has translated into a higher chance of destructive rains;14 parts of the US could experience a five-fold increase in the probability of extreme storms, which will also become more intense.15 Regional analyses are finding a decreasing trend in the wintertime snow- to-rain ratio.16
Global warming is also changing large-scale atmospheric circulation patterns,17 leading to increased snow and rain in some regions, increased risk of drought in other regions,18 and changes in monsoon patterns and monsoon weakening.19 It seems that wet areas are getting wetter and are more prone to flooding from heavy storms, while dry areas are getting drier.
Drought, fires, and food
Hotter temperatures dry out the soil more quickly, and when combined with lack of precipitation, produce drought. Drought is causing unprecedented wildfire in many regions of the world. In particular, drought is projected to worsen in the Southwest and Central Plains of the US over the coming decades.20
Due to a multiyear drought, 2015 was the worst fire year on record in the US. As of 2016, over 100 million trees had died due to California’s worst drought in over 1,200 years, which was driven by record temperatures.21 This unprecedented and worsening forest die-off might signal a permanent climate-driven ecologic shift.22
Climate change is now the primary driver for forest dryness and the longer fire season in the Western US, and has doubled the area that otherwise would have burned since 1984.23 Wildfire area increases exponentially with global warming, with each degree of warming leading to more fire than the last, at least while there are still trees left to burn.24
Drought also affects crop yields, as do heat waves. Although warming brings some benefits to high latitude crops, negative impacts on crop yields outweigh positive impacts globally, and warming is already reducing global wheat and maize yields.25
When temperature and precipitation regimes both change, there’s little in agriculture that isn’t affected. Here’s one example that might wake you up: by 2050, the area suitable for coffee production could be half as large as it was in 2016.26
Sea level
Coastal and low-lying areas have an increasing risk of flooding and submergence as sea levels continue to rise due to ice loss and thermal expansion.27 Sea level rise will not be globally uniform; some regions will experience more rise than others.28 Areas most at risk, in terms of overall cost of damage, include Miami, New York, New Orleans, Boston, Mumbai, and Guangzhou.29 These and other cities will eventually be forced to build costly protective structures, or risk abandonment; this could swamp the real estate market.30 Without adequate adaptation, hundreds of millions of people will be displaced by 2100.31
As this gradual sea level rise continues to accelerate, we also face additional, unpredictable increases due to ice sheet disintegration. For example, collapse of the West Antarctic ice sheet, which may now be inevitable due to past and ongoing destabilization, will raise sea level by at least 3 meters.32 But we don’t yet understand how the collapse of this and other ice sheets might unfold. Some models predict that West Antarctic collapse will take centuries.33 Another predicts decades, and the model underlying this faster prediction skillfully reproduces sea level rise in the geologic record.34 In the coming years, I’m certain that we’ll learn a great deal about ice sheet collapse, from both models and observations.
Human migrations and civil unrest
Global warming exacerbated a serious drought in Syria which helped precipitate civil war.35 While it’s important to keep in mind that warfare always arises from a mixture of factors, there’s nonetheless a clear link between climate change and increased risk of warfare,36 a risk that the Pentagon is taking very seriously.37
Pressures from sea level rise, drought, and famine due to global warming increase the risk of mass migration. Future climate-related migrations, for example from low-lying Bangladesh, could dwarf earlier refugee crises.
Socioeconomic distribution of impacts
How these impacts affect humans depends not only on warming, but on social structures. Financially poor and marginalized people are more vulnerable, and financially poor countries are less able to adapt and respond to climate crises.38 This fact, together with the fact that rich countries have emitted far more greenhouse gases per capita, adds a deep ethical dimension to our choice of response to global warming. This isn’t to say the rich are safe from these impacts. After enough warming, everyone will be vulnerable.
How long will global warming last?
The duration of global warming depends on how much CO2 we choose to emit, and how our emissions interact with the carbon cycle over time. Imagine we emit a pulse of CO2 into the atmosphere (it isn’t hard to do).
After a few years, the CO2 would become well-mixed in the atmosphere; and several processes in the carbon cycle would pull it from the atmosphere and into other carbon reservoirs on a variety of time scales: plant and soil uptake, ocean uptake, ocean floor sediment dissolution, and rock weathering.
As we saw in Chapter 3, plants and the ocean will remove roughly half of the emitted CO2 on a time scale of a few decades. What the plant and soil system will do on a longer time scale (say, a century) is a topic of active research: there is a good chance it will release some of the absorbed CO2 back into the atmosphere through fires and permafrost thawing.
The ocean will continue to absorb the CO2 on this century time scale. The precise fraction it absorbs depends on how much we emit. The more we emit, the higher the fraction of CO2 that will stay in the air (a warmer ocean holds less). The new equilibrium airborne fraction, achieved after a couple of centuries, will likely be between 15% and 40% and persist at this level for a few millennia. If we mitigate aggressively, it will be closer to 15%. If not, it will be closer to 40%.39
After this initial stage of rapid uptake by the ocean ends, the slower processes will take over. For the next few tens of thousands of years, calcite sediments on the seafloor will gradually dissolve in the acidified ocean, allowing it to draw more CO2 from the atmosphere until 10% or so of the CO2 remains. Then, for the next few hundreds of thousands of years, calcium carbonate precipitation in the ocean, driven by rock weathering on land, will finish the job.40
How can we predict that the ultimate time scale for CO2 recovery is a few hundred thousand years?
Aside from our models, which continue to improve, we have an extraordinary historical event with which to test them. The PETM was an abrupt global warming event that occurred about 56 million years ago. Its signature is clearly visible in ocean sediment cores.
Over the course of 8,000 years after the PETM, the Earth’s temperature rose by about 5°C to 8°C due to elevated CO2 in the atmosphere. (What triggered this elevated CO2 in the first place is still a mystery.) The CO2 fraction gradually recovered over the next 200,000 years.41
The PETM is the historical event most nearly matching today’s human-driven event. It’s the fastest climate change event we know of, except for today’s event—which is happening 10 to 100 times as rapidly, amplifying stresses to species and ecosystems.
Ten million years
It will take fifty or a hundred times this length of time, however, for biodiversity to recover. We know from fossil records that the biosphere takes ten million years to recover its biodiversity after mass extinctions.42 These two time scales—100,000 years for CO2 reuptake and 10 million years for biodiversity recovery—aren’t long from the Earth’s point of view. But they’re both good approximations to “forever” from the perspective of human lives or even human civilizations.
One of my main purposes in writing this book is to suggest that a good overarching goal for today’s civilization would be to minimize global warming and its concomitant biodiversity loss for the sake of the next few hundred thousand human generations.
At first, we didn’t know what we were doing. It was reason- able for us to start burning fossil fuels. However, now we do know what we’re doing. It bothers me to burn fossil fuels, and it also bothers most of my colleagues in Earth science.43 I’ve personally concluded that continuing to mindlessly burn fossil fuels, knowing what I know, is unacceptable.
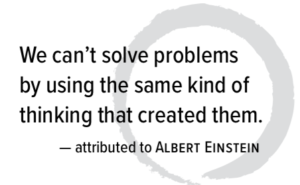
Geoengineering
Given the reality of global warming, those of us who judge it to be undesirable might reasonably hope that humanity will find a quick technological fix. This hope resonates with deep aspects of our modern industrial mindset, such as the fetishization of technology, the supremacy of convenience, and the desire to control nature. However, I currently see no evidence that geoengineering will save us; and in my opinion, it’s a mistake to hope that it will.
There are two broad categories of geoengineering ideas: those aiming to reflect sunlight and those aiming to take CO2 out of the atmosphere (carbon capture).
The leading carbon capture geoengineering technique,44 and the best idea I’ve seen so far, involves increasing the rate of weathering of silicate rocks by quarrying them, pulverizing them, and spreading them on fields in the tropics, where they would slowly absorb atmospheric CO2.45 But the scale of this operation would be massive, many times larger than the current scale of fossil fuel extraction.46 It would be extraordinarily expensive, require huge amounts of energy (either releasing yet more CO2 or requiring significantly more renewable energy infrastructure), and it could adversely affect soils.
Furthermore, we still don’t have a good understanding of weathering’s role in the carbon cycle: it’s unclear, for example, to what extent the long-term climate is controlled by the weathering of silicate rocks as opposed to carbonate rocks.47
It doesn’t make sense to expend huge amounts of energy and effort to take CO2 out of the atmosphere on the one hand, while we’re still releasing it by burning fossil fuels at an accelerating pace on the other. Perhaps someday we will implement large-scale renewable-energy-driven silicate weathering—after we’ve decarbonized our energy system.
The sunlight reflection approach could be implemented much more quickly and cheaply, but it has serious drawbacks. Members of the Royal Society (and others) consider airplane dispersal of sulfate aerosols into the stratosphere to be the “nearest approximation” to an “ideal method” of geoengineering.48 While this seems likely to lower global surface temperatures—we can’t be sure, because we don’t yet have a deep understanding of the albedo system, and our naïve attempts to engineer albedo might be buffered by compensating processes49—it would also carry great risks. No one knows all of the side effects it would impose on the Earth system, but global models suggest that it would lead to changes in the precipitation cycle, adversely affecting rainfall in some regions.50
This would create issues of international fairness. Certainly, wars have been fought for less; and the stakes of such water wars might be especially high, given the Indian subcontinent’s dependence on monsoon rains and the nuclear tensions there. Further, it’s fundamentally impossible for us to know all of the side effects in advance with models. The aerosol geoengineering option would amount to closing our eyes and jumping.
Another concern that I have, personally, is that we humans would collectively take the artificially lowered surface temperature as an excuse to continue postponing meaningful mitigation efforts. This combination of suppressed surface temperatures and lack of mitigation would leave the biosphere vulnerable to a sud- den temperature spike due to an unforeseen discontinuation of the geoengineering effort. For example, given the confluence of factors we’ll discuss in Chapter 5, it might be unwise to rule out the possibility of significant economic and political collapse. In the event of such a collapse, would the geoengineering planes keep flying? Steadily accelerating atmospheric accumulations of both CO2 and sulfate aerosol pollutants with the looming prospect of sudden cessation—what could go wrong?
Geoengineering prospects go downhill from here. Most other ideas, such as “space sunshades,” seem scarcely realistic or technologically realizable. For example, one proposal calls for “a swarm of trillions of thin metallic reflecting disks each about 50 cm in diameter, fabricated in space from near-Earth asteroids.”51
A better and easier idea, in my opinion, is to stop burning fossil fuels. It’s possible, as we’ll see in the second part of this book. The sooner we stop burning fossil fuels, the better off we’ll be.
Agricultural soil carbon sequestration
In Chapter 3, we discussed early experimental evidence that warm- ing will likely drive a net release of CO2 from global soils, mainly from Arctic and subarctic regions, although the magnitude of this release is still very uncertain. Keeping that in mind, what are the prospects for using soils to pull CO2 out of the atmosphere by adopting agricultural methods such as no-till, cover cropping, manuring, biochar, and improved grazing?
No one really knows. One study estimates uptake would be modest, perhaps offsetting 5% to 15% of global emissions annually;52 a more recent study puts the estimate at between 0% and 6%.53; This uptake would taper off until the soil reached a new equilibrium after between 20 and 100 years; the agricultural practices would then need to continue indefinitely to prevent rerelease back to the atmosphere.
Further research is needed in this area, but it would be a mis- take to expect “carbon farming” to be a silver bullet. The simple fact remains: we must stop burning fossil fuels.
How much more fossil fuel can we burn?
Scientists can imagine various mitigation pathways—particular mixes of technology, conservation, and human decisions—and then model emissions and temperature trajectories for these pathways.
The IPCC AR5 WG3 considered about 900 such pathways and translated them into actionable milestones. For example, to be on track for the RCP 4.5 scenario, with an estimated 66% chance at keeping peak warming under 3°C, at least 40% of humanity’s total energy supply must be carbon-free by 2050. To be on track for the RCP 2.6 scenario, with a 66% chance at keeping peak warming under 2°C, at least 60% of humanity’s total energy must be carbon- free by 2050.54
Unfortunately, the scenarios that would give us a fighting chance of remaining below 2°C aren’t realistic. Out of the 400 such scenarios, 344 assume large-scale deployment of negative- emission technologies such as carbon sequestration and rock- weathering geoengineering.55 The problem is that such technologies are speculative; they don’t yet exist. Also, each of the remaining 56 scenarios relies on global emissions peaking in 2010—but emissions didn’t peak in 2010, so these scenarios are already invalid. This kind of misplaced optimism does a disservice to humanity, because it lulls policymakers and the public into a false sense of security.
How much more fossil fuel, then, can we burn and maintain a decent chance of keeping warming below 2°C? For a 66% chance of staying under 2°C, we can emit a total of 270 [175 to 338] GtC from 2011 onward.<sup>56</sup> This includes everything: burning fossil fuels, burning forests, and making cement.
But emissions between 2002 and 2012 were 9.2 GtC per year on average, and growing exponentially at 2.2% per year. In just the five years from 2011 to 2016, therefore, we emitted at least another 46 GtC. Let’s optimistically assume that sometime between 2016 and 2100 we begin regrowing forests, and we do so well that there’s no net deforestation in this period; and that we constrain our cement emissions to 40 GtC from now until we phase out cement by 2100.57 This leaves 180 [89 to 252] GtC for burning fossil fuel. That’s our fossil fuel budget from 2016.
In 2010, we emitted 8.7 GtC from burning fossil fuels. At the 2.2% growth rate, we’ll burn through our budget by 2031 [2024 to 2036]. How quickly do we need to ramp down our emissions to stay within budget?
Suppose we’d started in 2016. We’d have needed to ramp down by 6% per year (or 12% per year in the worst-case scenario, where the 2016 budget turned out to be only 89 GtC). And the longer we wait, the more quickly we’ll need to ramp down. If we wait until 2020, we’d then need to reduce by at least 10% per year (or 40% per year in the worst-case scenario).
Any way you slice it, keeping warming below 2°C requires an immediate, massive, and global mitigation effort. With each passing day, it’s less likely that we’ll succeed. Indeed, over 90% of Earth scientists believe we’ll surpass this threshold.58
Clearly, production of unconventional resources such as the Alberta tar sands or Arctic oil is not consistent with the 2°C goal. If we need to leave huge fractions of our fossil fuel reserves in the ground, the rational response would be to immediately give up exploration and development of these less economic resources, which we won’t need if we’re indeed planning to ramp down.59 That we’re in fact pursuing them is not cause for optimism.
I hope this discussion has given a clear and informed sense of urgency.
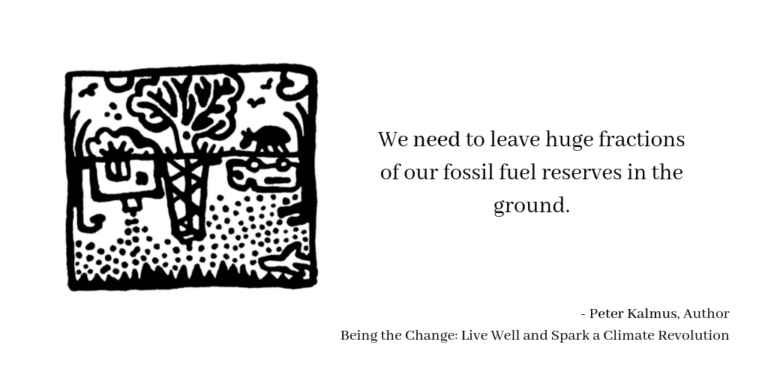
Causes for optimism
Despite this urgency, I feel optimistic about meaningful collective action on several fronts. One is renewable electricity, which is growing rapidly. One hundred percent carbon-free electricity is within reach in the US; I estimate that we could make our entire electrical grid carbon-free for a few thousand dollars per person, far less than we’ve already spent on the war on terror.60 Of course, US electricity is only part of a much bigger picture which includes food, forestry, development, manufacturing, transportation—and the world outside of the US. But by 2016, one-quarter of global electricity was generated renewably.61
Another is California, my home state and arguably the world’s 6th-largest economy, which is on track to be 40% below 1990 emissions levels by 2030.62 California is actually doing something: setting meaningful goals and achieving them. Other states and countries should do the same.
A third is the prospect of the US someday adopting a national revenue-neutral carbon fee (see Chapter 14), which would greatly accelerate the transition to a carbon-free energy system and cause this transition to pervade every aspect of our lives. When the US adopts a revenue-neutral carbon fee, other nations would likely follow suit.
Uncertainty
In this and the previous chapter, I’ve attempted to point out which scientific details are certain and which are still uncertain. However, it’s a mistake to think that successful collective action depends on reducing the scientific uncertainty surrounding some of the details of global warming. For one thing, the overall scientific picture is unequivocal63—continuing on our current path will lead to catastrophic changes to the Earth system that gives us life. It is this overall picture which demands action.
The remaining scientific uncertainties are now overshadowed by cultural, economic, and political uncertainties. Indeed, the largest uncertainty of all is how much fossil fuels humans will choose to burn.
We climate scientists have done good work. We’ve concluded, unequivocally, that global warming is caused by humans burning fossil fuels, although we continue to understate the urgency and seriousness of the threat by systematically “erring on the side of least drama.”64 Although we’d do well to learn how to connect more effectively with the public—to become storytellers—we have nonetheless delivered our message. How humanity acts on that message is up to the policymakers, which really just means it’s up to people like you.
- For a more complete discussion of impacts, please see the global warming books I listed in Chapter 3, note 2, or the IPCC AR5 WG2 report.
- IPCC AR5 WG1 SPM.
- Jean-Marie Robine et al. “Death toll exceeded 70,000 in Europe during the summer of 2003.” Comptes Rendus Biologies 331 (2) (2008). [online]. doi:10.1016/j.crvi.2007.12.001.
- Peter A. Stott et al. “Human contribution to the European heatwave of 2003.” Nature 432 (2004). [online]. doi:10.1038/nature03089.
- Nikolaos Christidis et al. “Dramatically increasing chance of ex- tremely hot summers since the 2003 European heatwave.” Nature Climate Change 5 (2015). [online]. doi:10.1038/nclimate2468.
- I-Ching Chen et al. “Rapid range shifts of species associated with high levels of climate warming.” Science 333(6045) (2011). [online]. doi:10.1126/science.1206432; Kai Zhu et al. “Failure to migrate: Lack of tree range expansion in response to climate change.” Global Change Biology 18(3) (2011). [online]. doi:10.1111/j.1365-2486.2011.02571.x; Harald Pauli et al. “Recent plant diversity changes on Europe’s mountain summits.” Science 336(6079) (2012). [online]. doi:10.1126 /science.1219033.
- Elvira S. Poloczanska et al. “Global imprint of climate change on marine life.” Nature Climate Change 3 (2013). [online]. doi:10.1038 /nclimate1958.
- Chris D. Thomas et al. “Extinction risk from climate change.” Nature 427 (2004). [online]. doi:10.1038/nature02121.
- Francesca A. McInerney and Scott L. Wing. “The Paleocene-Eocene Thermal Maximum: A Perturbation of Carbon Cycle, Climate, and Biosphere with Implications for the Future.” Annual Review of Earth and Planetary Sciences 39 (2011). [online]. doi:10.1146/annurev-earth -040610-133431.
- E. O. Wilson. Half-Earth: Our Planet’s Fight for Life. Liveright, 2016, p. 14.
- Mark C. Urban. “Accelerating extinction risk from climate change.” Science 348(6234) (2015). [online]. doi:10.1126/science.aaa4984.
- Mora et al. “The projected timing of climate departure.”
- IPCC AR5 WG1 SPM.
- Ibid.
- Andreas F. Prein et al. “The future intensification of hourly precipitation extremes.” Nature Climate Change 7 (2017). [online]. doi:10.1038/nclimate3168.
- IPCC AR5 WG1 Chapter 2, p. 204.
- IPCC AR5 WG1 Chapter 2, p. 223.
- At the time of this writing, a definitive global warming attribution for specific droughts has not been made, but evidence is mounting.
- IPCC AR5 WG1 Chapter 2, p. 227.
- Benjamin I. Cook, Toby R. Ault, and Jason E. Smerdon. “Unprecedented 21st century drought risk in the American Southwest and Central Plains.” Science Advances 1(1) (2015). [online]. doi:10.1126 /sciadv.1400082.
- Daniel Griffin and Kevin J. Anchukaitis. “How unusual is the 2012– 2014 California drought?” Geophysical Research Letters 41 (2014). [online]. doi:10.1002/2014GL062433.
- Mike McPhate. “California today: More than 100 million trees
are dead. What now?” New York Times, November 21, 2016. [online]. nytimes.com/2016/11/21/us/california-today-dead-trees-forests.html. - John T. Abatzoglou and A. Park Williams. “Impact of anthropogenic climate change on wildfire across western US forests.” Proceedings of the National Academy of Sciences 113(42) (2016). [online]. doi:10.1073 /pnas.1607171113.
- Tatiana Schlossberg. “Climate change blamed for half of increased fire danger.” New York Times, October 10, 2016. [online]. nytimes.com /2016/10/11/science/climate-change-forest-fires.html.
- IPCC AR5 WG2 SPM.
- Corey Watts. “A brewing storm: The climate change risks to coffee.”Climate Institute, 2016. [online]. climateinstitute.org.au/coffee.html. Climate-related threats to the global coffee crop include drought, rising temperatures, and increases in pests and disease.
- IPCC AR5 WG2 Chapter 5, p. 364.
- Ibid.
- Stephane Hallegatte et al. “Future flood losses in major coastal cities.” Nature Climate Change 3 (2013). [online]. doi:10.1038/nclimate1979.
- Ian Urbina. “Perils of climate change could swamp coastal real estate.” New York Times, November 24, 2016. [online]. nytimes.com/2016/11/24/science/global-warming-coastal-real-estate.html.
- IPCC AR5 WG2 Chapter 5, p. 364.
- Eric Rignot et al. “Widespread, rapid grounding line retreat of Pine Island, Thwaites, Smith, and Kohler glaciers, West Antarc- tica, from 1992 to 2011.” Geophysical Research Letters 41(10) (2014). [online]. doi:10.1002/2014GL060140; Johannes Feldmann and Anders Levermann. “Collapse of the West Antarctic Ice Sheet after local destabilization of the Amundsen Basin.” Proceedings of the National Academy of Sciences 112(46) (2015). [online]. doi:10.1073/ pnas.1512482112.
- Feldmann and Levermann. “Collapse of the West Antarctic Ice Sheet after local destabilization of the Amundsen Basin.” Ian Joughin et al. “Marine ice sheet collapse potentially under way for the Thwaites Glacier Basin, West Antarctica.” Science 344(6184) (2014). [online]. doi:10.1126/science.1249055.
- Robert M. DeConto and David Pollard. “Contribution of Antarc- tica to past and future sea-level rise.” Nature 531 (2016). [online]. doi:10.1038/nature17145.
- Colin P. Kelley et al. “Climate change in the Fertile Crescent and implications of the recent Syrian drought.” Publications of the Na- tional Academy of Sciences 112(11) (2015). [online]. doi:10.1073/pnas .1421533112; John Wendle. “The ominous story of Syria’s climate refugees.” Scientific American, December 17, 2015. [online]. scientific american.com/article/ominous-story-of-syria-climate-refugees/.
- Solomon M. Hsiang et al. “Quantifying the influence of climate on human conflict.” Science 341(6151) (2013). [online]. doi:10.1126 /science.1235367.
- W. J. Hennigan. “Climate change is real: Just ask the Pentagon.” Los Angeles Times, November 11, 2016. [online]. latimes.com/nation/la-na -military-climate-change-20161103-story.html.
- IPCC AR5 WG2 SPM.
- IPCC AR5 WG1 Chapter 6, p. 544.
- Archer. The Global Carbon Cycle.
- McInerney and Wing. “The Paleocene-Eocene Thermal Maximum.”
- James W. Kirchner and Anne Weil. “Delayed biological recovery from extinctions throughout the fossil record.” Nature 404 (2000). [online]. doi:10.1038/35004564.
- In the survey of Earth scientists I mentioned in Chapter 3, when asked “Does it bother you, personally, to burn fossil fuels?” 52% said “yes,” 41% said “sometimes,” and 7% said “no” (58 responses).
- According to the Royal Society: Geoengineering the climate: Science, governance and uncertainty. RS Policy document 10/09, 2009. [online]. royalsociety.org/topics-policy/publications/2009/geo engineering-climate/.
- Lyla L. Taylor et al. “Enhanced weathering strategies for stabilizing climate and averting ocean acidification.” Nature Climate Change 6 (2016). [online]. doi:10.1038/nclimate2882.
- It takes about two tonnes of silicate rock to remove one tonne of CO2. But one tonne of carbon mined from the ground releases about four tonnes of CO2 gas when burned. So every tonne of carbon mined would require about eight tonnes of silicate rock to mitigate.
- Ziahua Liu et al. “Atmospheric CO2 sink: Silicate weathering or carbonate weathering?” Applied Geochemistry Vol. 26 (Supplement) (2011). [online]. doi: dx.doi.org/10.1016/j.apgeochem.2011.03.085.
- Royal Society. Geoengineering the climate, p. 49.
- Graeme L. Stephens et al. “The albedo of Earth.” Reviews of Geophysics 53 (2015). [online]. doi:10.1002/2014RG000449.
- H. Damon Matthews and Ken Caldeira. “Transient climate- carbon simulations of planetary geoengineering.” Publications of the National Academy of Sciences 104(24) (2007). [online]. doi: 10.1073pnas.0700419104.
- Royal Society. Geoengineering the climate, p. 32.
- R. Lal. “Soil carbon sequestration impacts on global climate change and food security.” Science 304(5677) (2004). [online]. doi:10.1126 /science.1097396.
- David S. Powlson et al. “Limited potential of no-till agriculture for climate change mitigation.” Nature Climate Change 4 (2014). [online]. doi:10.1038/NCLIMATE2292.
- IPCC AR5 WG3 SPM, Figure SPM.4 and Table SPM.1. These requirements are based on the median pathways presented for each scenario.
- Kevin Anderson. “Duality in climate science.” Nature Geoscience 8 (2015). [online]. doi:10.1038/ngeo2559.
- IPCC AR5 synthesis report SPM. The total estimated budget from 1870 onward is 790 [695 to 858] GtC, and 520 GtC was emitted up to 2011.
- Anderson. “Duality in climate science.”
- In my survey of Earth scientists, when asked “Do you believe that we will stay below the 2 degree Celsius threshold?” 92% said “no” while 8% said “yes” (60 responses).
- Christophe McGlade and Paul Ekins. “The geographical distribution of fossil fuels unused when limiting global warming to 2°C.” Nature 517 (2015). [online]. doi:10.1038/nature14016.
- I looked at current costs of wind, solar voltaic, concentrating solar trough, and nuclear energy, and electrical storage; made some basic assumptions about energy storage; and assumed that as a nation we can cut our electricity use in half. This led to an estimate that electrical decarbonization would cost about $1 trillion. This is about $3,000 per person, or alternatively the combined net worth of the twenty or so richest Americans. By comparison, by 2015, the “war on terror” had cost US taxpayers $1.7 trillion: Niall McCarthy. “The war on terror has cost taxpayers $1.7 trillion.’’ Forbes, February 3 2015. [online]. forbes.com/sites/niallmccarthy/2015/02/03/the-war-on -terror-has-cost-taxpayers-1-7-trillion-infographic/#75a6f1255cf0.
- Three quarters of this was from hydropower. World Energy Council. “Variable renewable energy sources integration in electricity systems 2016: How to get it right.” (2016). [online]. worldenergy.org /publications/2016/variable-renewable-energy-sources-integration -in-electricity-systems-2016-how-to-get-it-right/.
- Kanyakrit Vongkiatkajorn. “California just took a huge step in the fight against climate change.” Mother Jones, September 8, 2016. [online]. motherjones.com/environment/2016/09/california-passes -sb-32-groundbreaking-climate-legislation.
- It’s important to realize that no scientist will say he or she is 100% certain about any future outcome, ever. No one can be 100% certain about any event in the future. There should be a word in our language which scientists can use to mean “certain beyond any reasonable doubt” and which non-scientists hear as “absolutely certain.” I’ve chosen to use the word “unequivocal” for this purpose.
- Keynyn Brysse et al. “Climate change prediction: Erring on the side of least drama?” Global Environmental Change 23(1) (2013). [online]. doi:10.1016/j.gloenvcha.2012.10.008.